The offshore wind industry needs to substantially increase the durability, strength, and environmental sustainability of its wind-turbine-generator support structures while reducing the carbon emissions associated with construction materials and processes. The changes proposed below are not only environmentally ethical, but fiscally responsible, and these long-term benefits become even more significant as future offshore wind projects scale up in volume and size.
Offshore wind turbine generator support structure designs
The designs of wind-turbine generator (WTG) support structures planned for offshore use on both the East and West coasts of the United States have now been standardized. On the East Coast, two types of fixed bottom turbine support structures will be used on the continental shelf: monopile (a large pipe) and jackets (a steel lattice). Approximately 80 percent of the leases are planned to be monopile, and the remainder will be jackets. On the West Coast, 100 percent of projects will be floating support structures and will use either a spar buoy (a long, large tube) or a semi-submersible (three large vertical pipe tanks fixed to each point of a large triangle).
Unfortunately, the construction and deployment of these current designs have yet to incorporate production and construction processes, which can greatly reduce carbon emissions, nor do they integrate strong, environmentally sustainable materials necessary to achieve long-lived structures capable of successfully surviving multiple generations in a harsh marine environment.
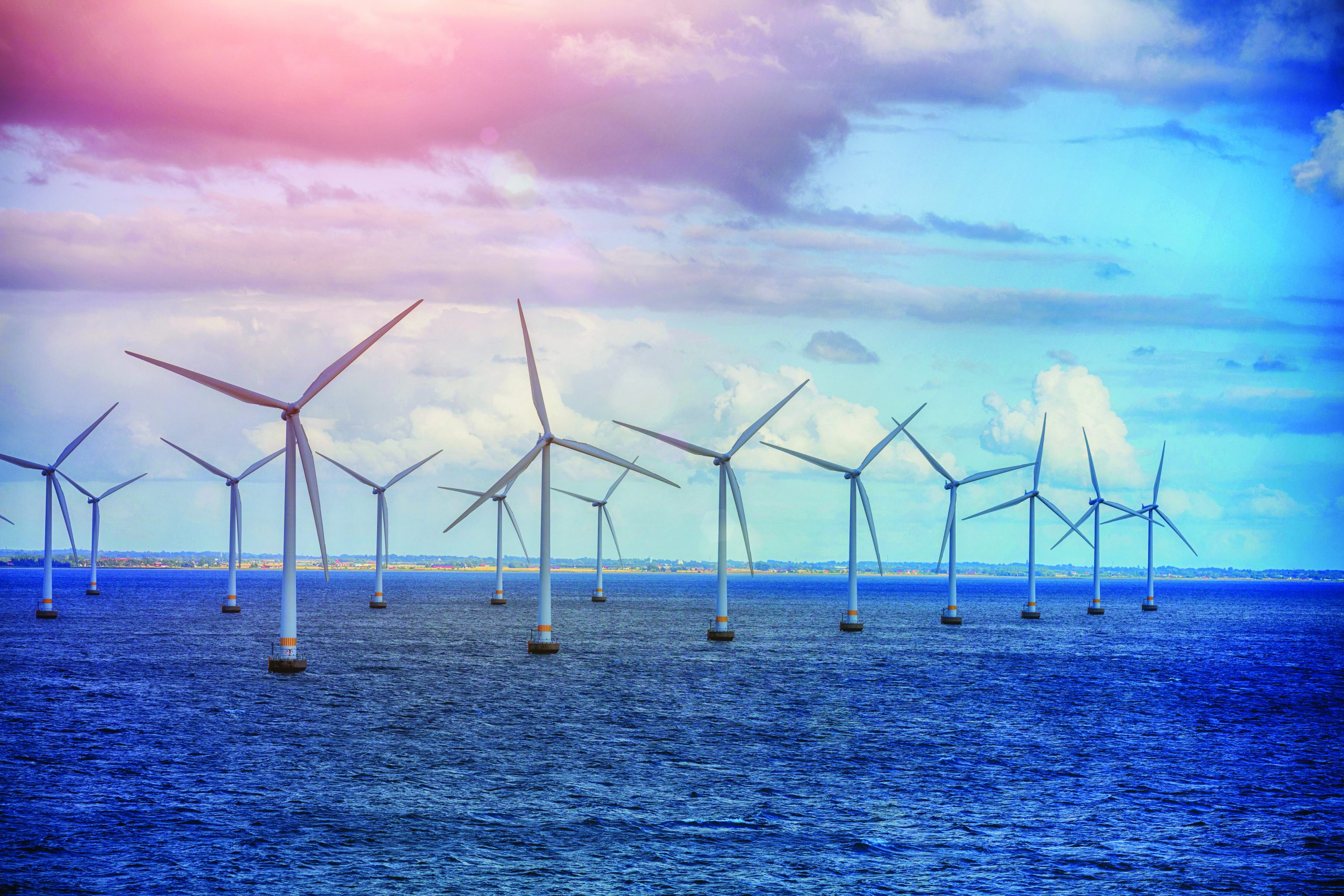
Obsolete materials used in offshore structures
Current structures made from steel are heavy and require a long production process. Steel production is responsible for generating 7 percent of all carbon dioxide emissions worldwide. More than 70 percent of steel production is accomplished using century-old blast furnace technology whereby coal is burned at a high temperature to reduce the oxygen in iron ore, which is then eventually turned into steel.
According to the International Energy Agency (IEA), all the steel currently used for the construction of offshore WTG support structures originates from coal-fired plants in Poland, Spain, and Russia. The only “green” steel in Europe is produced in Sweden using hydrogen, but this steel is used in truck construction and has not yet been made available for wind projects.
One-hundred percent of the concrete used in current floating structures is made using ordinary Portland cement (OPC). The OPC (binder) is the paste that holds traditional concrete together, but it has deficiencies when used in marine environments. Salt water directly attacks the chemistry of OPC, causing rapid failure. Sulfur compounds in sea water directly attack the calcium components of OPC, and this chemical reaction essentially “rots” the concrete. OPC concrete needs to be heavier in its structural volume in seawater applications since it requires more cover to protect the steel rebar.
New lighter green materials are available for offshore wind
The steel, rebar, and OPC concrete used in the construction of offshore wind-turbine-generator support structures can easily be replaced with lighter, stronger materials with a substantially smaller carbon footprint. One of these greener materials is geopolymer concrete. Geopolymer binders are used all over the world due to their superior performance to ordinary Portland cement (OPC) binders. They are stronger, fireproof, and waterproof. They bond well to most materials, have minimal expansion or contraction, are formable, and are resistant to salt, acids, and alkalis. The production process for geopolymer cement has an 80 percent smaller carbon footprint than OPC.
Steel rebar reinforcement can be replaced with a nonmetallic bar made from readily available basalt stone. Basalt stone Is found all over the Earth and is one of the key components for durable offshore construction in a marine environment. Basalt stone, when heated to a temperature of 1,800 degrees Fahrenheit, liquifies and can be run through a palladium die that produces soft, flexible threads. The threads are laid in parallel and locked together with an epoxy, producing basalt rebar.
Compared to a similar diameter steel rebar, basalt rebar is seven-to-nine times lighter while providing the same strength. Since basalt is stone, there is no cover requirement; it is waterproof, chemically resistant, and fireproof. The tensile strength of basalt rebar is three times stronger than steel rebar. Basalt rebar is extremely light and relatively flexible, leading to easy placement in the structure. Cut-basalt fiber additives, much like nylon fiber, are used in the concrete mix design for added strength. The geopolymer cement in the concrete binds to the basalt rebar both chemically and mechanically. Due to the low porosity, high strength, and chemically resistant nature of geopolymer cement and basalt rebar, offshore wind substructures constructed with these materials will have a minimum of a 100-year life.
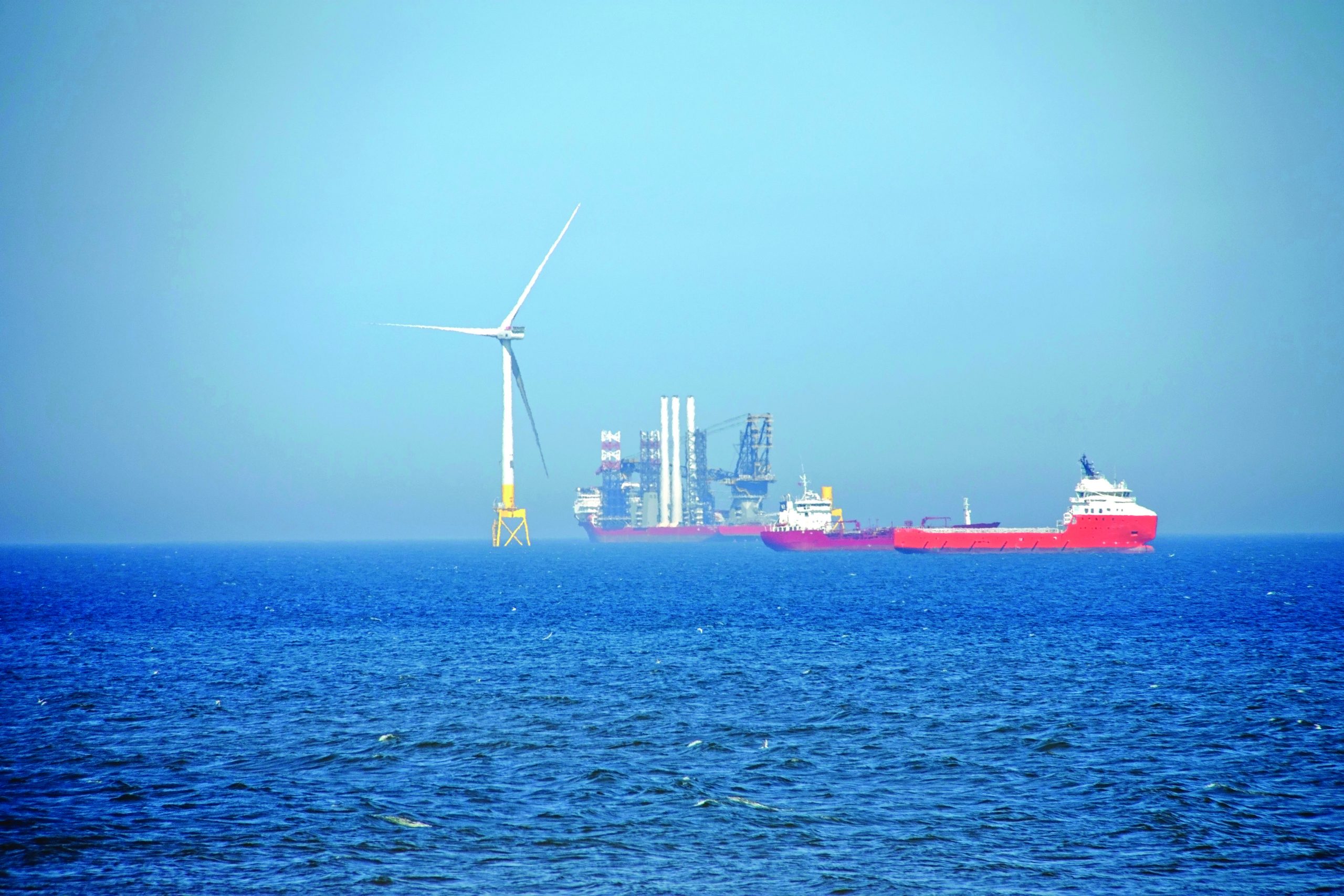
Electrical curing of geopolymer concrete
Although a small amount of custom formwork will be used for forming small add-on components, most of the construction of the offshore wind structures can be achieved using slip-form technology. Electrical curing adds an additional layer of efficiency to the slip-forming process. When the concrete mix design is plastic, the mix has an elevated conductivity because it hasn’t yet polymerized. When a direct or alternating current is passed through the concrete, it begins to set up immediately. Heat is being generated (above 85 degrees Fahrenheit), and the current is relatively small.
After the concrete has set, which is a few seconds after the initial charge, the charge can be increased to expedite the strength gain. Heat Is an important factor in the strength gain experienced during electrical curing. Electrical curing of the concrete speeds up the slip form process (two feet/hour rather than six inches/hour), resulting in an overall reduction in concrete slip time by 75 percent along with the associated huge reduction in labor costs.
Reducing weight and cost
The tower structure for a 15-MW direct drive wind turbine generator (WTG) weighs well over 1,000 tons and has a lifespan of 25 years. Using new green materials reduces the tower weight to only 350 tons while increasing the lifespan to a minimum of 100 years. Using the slip-form construction process for the tower creates the opportunity to slip-form the tower sections in the back harbor, thereby eliminating the significant freight costs associated with transporting a tower from an off-site plant to the wind-farm location. After installation of internals, the tower could be ready in just two weeks, resulting in yet another reduction in labor costs. The 650-ton reduction of the weight of the tower will reduce the hull displacement, saving approximately 600 tons of materials.
Greening of monopile support structure
The fixed-bottom monopile, supporting the majority of the forthcoming offshore WTGs on the East Coast, is a prime candidate for construction with geopolymer cement and basalt rebar. By slip-forming the monopile on a construction vessel outside the harbor, a 40-foot diameter, 400-foot-long monopile could be produced every nine days (with floater end caps) ready for self-float transport. Once the monopile is in place, a transition component — known as the transition platform — slips over the exposed end of the monopile, is used for leveling the turbine platform, and is grouted to the monopile with more geopolymer binder. This transition platform is a slip-formed component and includes cemented add-ons such as the turbine interface platform, concrete handrails, intermediate platforms, concrete ladders, J-tubes, and boat-bumper attachments.
All these add-ons are formed from custom formwork and will be attached to the transition platform with geopolymer binder. Since this geopolymer bonding is both mechanical and chemical, there is no cold shot.
A slip-formed geopolymer concrete monopile of this size would have an overall weight of 850 tons. An identical steel monopile would weigh three times as much with a weight closer to 2,500 tons. Fabricating a steel monopile requires a plant outfitted with high-capacity overhead cranes and high-capacity floors. The transport requirements for a steel monopile are complex and expensive including up to 40 dollies to move the monopile out of the plant and high-capacity cranes to load the monopile on a transport barge for deployment to its station-keeping location in the farm. Once in place, a large jack-up vessel with a high-capacity crane is used for upending and placement (also known as a bottom-supported platform) to drill, drive, or vibrate the steel monopile to grade. These processes increase an already large carbon footprint.
Green station-keeping anchors for floating systems
Based on sea floor conditions, one of two types of anchor systems can be used for floating wind systems. To coincide with the 100-year lifespan of the geopolymer cement and basalt rebar WTG support structures, each anchor system will be constructed of these same sustainable materials. Using these new materials will reduce the capital cost associated with traditional mooring and anchoring. The first system, a suction caisson anchor, is good for resisting high loads. The anchor resembles an overturned bucket placed on the sea floor where water is pumped out creating a vacuum, which pulls the anchor down into the seabed. These suction anchors, planned for use with large 15- to 20-MW floating WTGs, will have a 26 foot outside diameter and will be 72 feet long. It will be built top-down with custom formwork, then the tube section will be slip-formed.
The second type of anchor is a geopolymer concrete chain. Each custom molded link is eight feet long with a link thickness of a foot and a half. This concrete chain is laid on the sea floor perpendicular to the center line of the float. It acts as a gravity anchor with only one of its many links rarely lifting off the sea floor. It works well on sea floors that will not accept a suction caisson.
A huge reduction in decommissioning costs
To date, the majority of offshore wind developers have sold their wind-farm leases to new major energy companies. Some of these energy companies are wearing the developer’s hat as well. All offshore wind leases now are based on the material life of the WTG, which is currently structured at a 25-year maximum. The U.S. government requires offshore wind installation lease holders to provide financial security to ensure decommissioning obligations are carried out. A bond is required, prior to construction, covering the estimated decommissioning cost, which ranges from 60 to 70 percent of estimated capital cost.
Overall project budget development will require a report specifying how the decommissioning will be carried out. The process is normally a reversal of the deployment installation.
Although the nacelle and blades will still need to be replaced every 25 years, the new generation of green materials outlined here can produce offshore WTG towers and foundation structures with a minimum lifespan of 100 years. These new structures can support four generations of wind turbines and significantly reduced decommissioning costs. The process for changing out the turbine nacelle and blade could be as little as two days per turbine. New large international renewable energy companies are bidding on and buying up offshore wind leases around the world. The new bid requirements for contract developers should be a minimum 100-year lifespan requirement in their structural materials and leases to avoid tearing down a multimillion-dollar offshore windfarm every 25 years.
Environmental sustainability results on fiscal responsibility
Reducing the environmental impact associated with offshore wind systems should be a paramount goal, but money is often a limiting factor. Not only do the materials and processes discussed significantly decrease the carbon footprint of an offshore wind farm, but an outside cost opinion found there would be a 32-percent reduction in the cost of materials and a 28-percent reduction in the cost of labor for construction and development.
The 100-year lifespan of the support structures will eliminate the multimillion-dollar cost of purchasing, constructing, deploying, and decommissioning offshore windfarms every 25 years.