The offshore wind industry has yet to reach its full potential. But, over the last 10 years, innovative designs and advanced technology has lowered the levelized cost of energy (LCOE). Even with this rapid progress, offshore wind is still in its infancy as a go-to energy production and consumption source.
Recent advancements have allowed wind developers to literally venture into deeper waters. Farther offshore, we can harness the full potential of wind and use it to bring clean energy to transmission grids in regions worldwide.
Some already have begun to see the monetary, environmental, and ecological benefits floating wind farms in waters deeper than 60 meters can provide — especially when compared to bottom-fixed offshore wind structures. To realize its full potential, wind developers need to address several performance-related issues so offshore wind components in deeper waters obtain cost-effective designs that achieve favorable performance while maintaining structural integrity.
From top to bottom, how can floating offshore developers make the most of their wind farms?

Performance of Turbines and Towers
According to the International Renewable Energy Agency (IRENA), the average size of offshore wind turbines grew by a factor of 3.4 in less than two decades and is expected to grow to an output capacity of 15 to 20 MW by 2030.
Modern wind turbines are becoming more cost-effective and reliable over time, even with this steady increase in size and output. Increased cost-effectiveness and reliability are due to the more efficient use of steel, iron, and other materials like fiberglass used to produce them. The widespread adoption of 3D printing and modeling also reduces the time and labor-intensive process of constructing blades and turbine components. With fewer input materials and less manual labor required, implementing 3D printing and automation can easily allow developers to create component molds and turbine components quickly.
Software innovations also allow computer modeling, simulations, and data analysis to become a prevalent part of development, design, and construction. Software can be used to construct visual models and perform simulations to test performance in several different conditions while applying numerous external and internal factors. These tools allow for more thorough system analyses of every part of the turbine to better harness wind.
New technologies also allow developers to actively measure, model, and analyze how wind turbines interact with the grid. Retooling their concepts allows developers another opportunity to rethink the design and implementation of turbines before scaling up and increasing the number of units in a given wind farm.
While many wind developers are already using some or all of these tools and technologies in their design and manufacture of turbines, the standardization of these techniques will be critical to implementing more innovative, efficient, optimized designs.

Performance of Platforms and Mooring Systems
The most prominent factors in increasing offshore wind performance are the structures and systems supporting the turbines and towers. This is especially true for floating wind developers.
At depths greater than 60 meters, it becomes harder to build, secure, and maintain fixed platforms to the seafloor. Fixed structures also need more steel and concrete as they increase in size. The immediate solution to support larger towers and turbines are larger platforms, requiring more steel and/or concrete. Because of this, fixed platforms are harder to secure and less cost-effective in deeper waters.
Floating platforms remain the most viable solution to take full advantage of more robust, less intermittent winds farther from shore. However, the approach for reducing the load on the tower and turbine and decreasing the pitching motion associated with increased wave activity in rougher conditions is still a subject of debate.
The three most common floating platform concepts in the offshore wind industry are spar-buoy, tension-leg platform, and semi-submersible. Although there are additional concepts in the design and development phase, most designs are variations of these three concepts.
Developers should consider the pros and cons of these platforms before trying to implement a completely new design. It is essential to ask these questions:
- What do these designs do well?
- What factors affect their performance the most?
- How do their mooring systems factor into their efficiency?
- How are they constructed?
- How are they transported?
- How easy are they to maintain and repair?
Semi-submersible designs can be constructed onshore or in shipping yards and docks and transported to sites using conventional tugs. Still, they tend to use more steel and concrete than other floating designs and often have higher critical wave-induced motions.
Tension-leg platforms do not have the same problems with wave-induced motions.
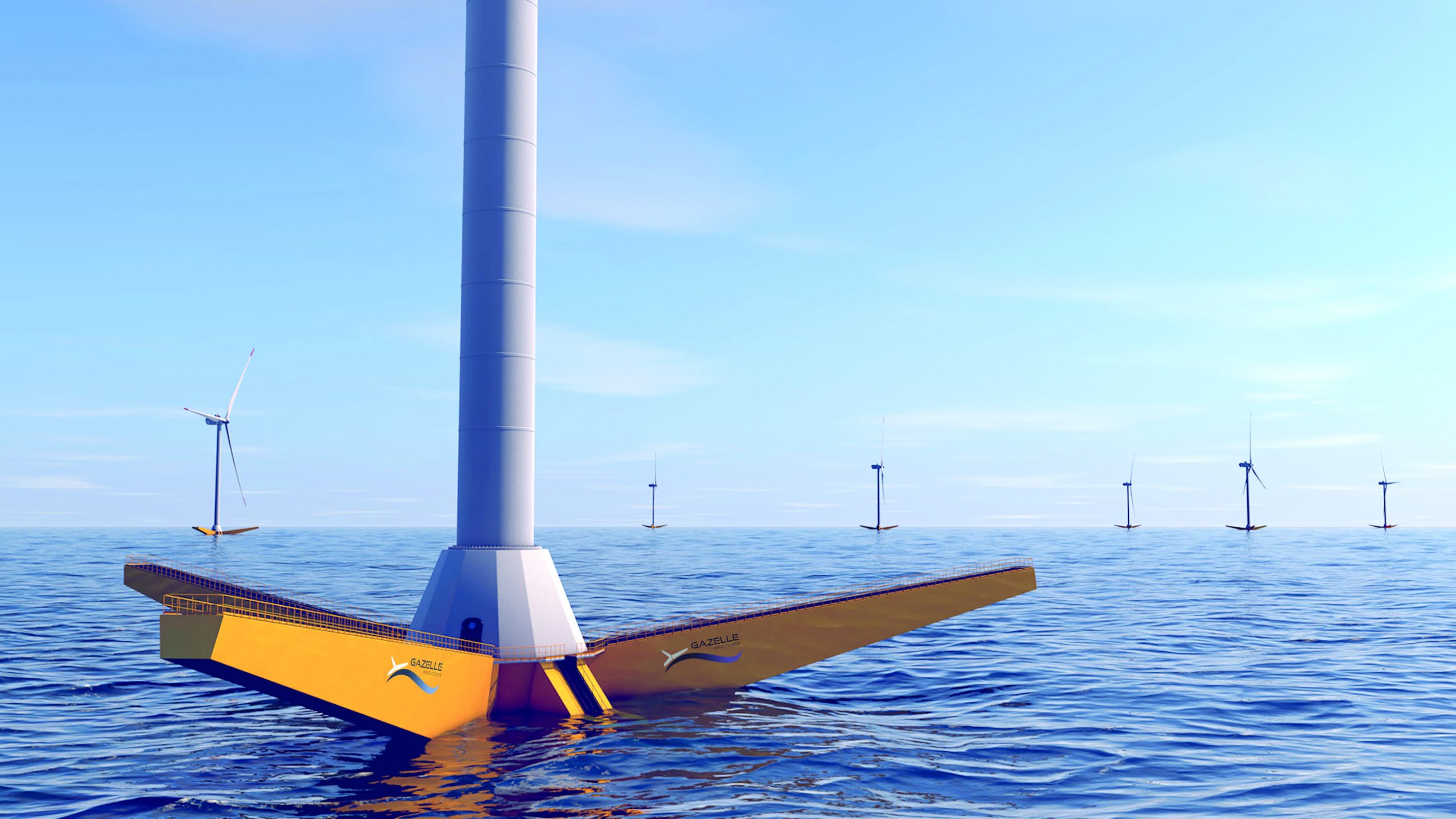
Nevertheless, because they are highly buoyant, they can be difficult to keep stable during transport and installation — and also have a higher installed mooring cost.
Spar-buoy designs have a simple design and lower mooring cost, but they require heavy-lift vessels to put them in place and often cannot withstand rough conditions in waters between 60 and 100 meters.
The key to using the best of all of these concepts, without their drawbacks, goes back to an old adage: “Sometimes, less is more.”
Putting costs aside, larger structures that require more steel and concrete are not the answer. They are worse for the environment and require pontoons and specialized mooring systems to keep them buoyant. And even still, added buoyancy does not ensure optimal performance.
A hybrid floating platform that combines the best features of tension-leg and semi-submersible platforms eliminates some of the drawbacks of other floating wind platform concepts while allowing wind farms to be placed in deeper waters (potentially 400 meters or more). They also allow the platforms themselves to move vertically and horizontally with the wind and the waves. Rethinking the platform and mooring system designs could dramatically decrease the tonnage of steel and concrete needed by as much as 70 percent, allowing for lighter, more reliable structures.
Hybrid platform designs that use fewer input materials allow the platform to move with almost zero-pitch angle. Null pitch means reduced wearing, less maintenance, and ultimately leads to longer life of the turbine.
At greater depths, floaters need their own individual mooring systems. The greater the length or number of mooring lines each system has, the greater the chance that those lines fail. This could prove catastrophic for every unit in a given wind farm. Designs that use fewer mooring lines would allow for increased performance. Hybrid designs could use up to 80 percent fewer mooring lines (maximum loads) than standard tension-leg structures and 75 percent fewer mooring lines (length) than conventional semi-submersible designs.
Revamped mooring systems will also reduce loads — which ultimately reduces the size and costs of foundations and installation. It is possible to reduce horizontal movements of floating platforms by as much as 70 percent. Smaller horizontal movements mean smaller swept areas and less drift than many current designs.

These lighter, smaller, efficient designs will allow for easier installation and commissioning — meaning standard-sized harbors, tugs, and cranes can all be used to construct all of the components and transport them to the site of the wind farm — with the potential to produce savings as much as 1 million euros per megawatt.
Implementing designs that address improvements to the turbines, platforms, and mooring systems will lead to increased effectiveness and longevity, which allows for less maintenance — meaning less risk and greater safety for the workers maintaining and repairing them. Additionally, turbine, platform, and mooring components in smaller, lighter structures can be brought into the harbor or shipyard for repairs and replacements, creating greater efficiency.
Final Thoughts
Increased efficiency will come with time as new technology will allow for a deeper scientific analysis on the performance of mooring systems, platforms, turbines, and other components — but developers should constantly be studying and rethinking their designs so the implementation of cleaner energy for a growing worldwide population can continue to accelerate.